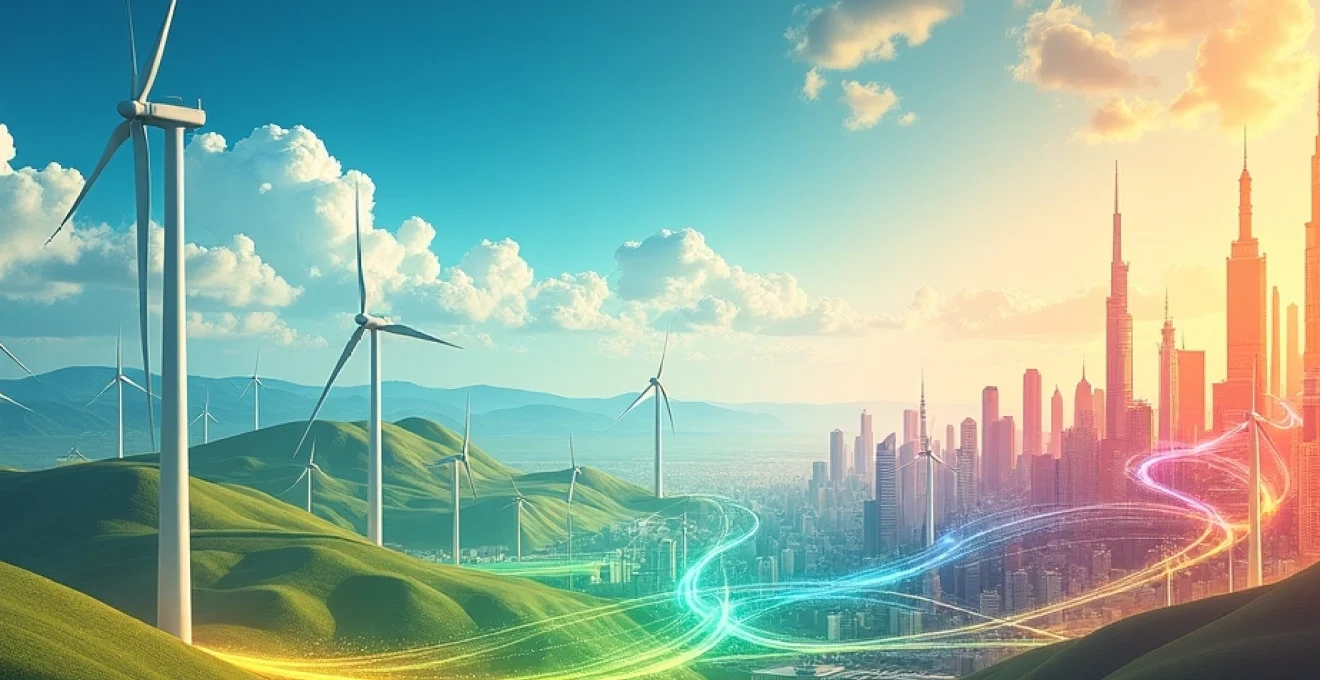
Wind farms have emerged as a cornerstone of sustainable energy production, revolutionizing our approach to power generation and grid management. As the world increasingly turns to renewable sources, wind energy stands out for its scalability, efficiency and minimal environmental impact.
Wind farm technology and infrastructure
The technological advancements in wind farm design and infrastructure have been nothing short of remarkable. These innovations have dramatically increased the efficiency and output of wind turbines, making wind energy an increasingly viable and cost-effective option for large-scale power generation.
Horizontal Axis Wind Rurbines (HAWT) vs. Vertical Axis Wind Turbines (VAWT)
When it comes to wind turbine design, two main types dominate the landscape: Horizontal Axis Wind Turbines (HAWT) and Vertical Axis Wind Turbines (VAWT). HAWTs are the most common type, characterized by their propeller-like blades that rotate around a horizontal axis. These turbines are highly efficient in areas with consistent wind direction and are the preferred choice for large-scale wind farms.
On the other hand, VAWTs rotate around a vertical axis, making them less dependent on wind direction. While they're generally less efficient than HAWTs, they offer advantages in urban environments or areas with turbulent wind conditions. The choice between HAWT and VAWT depends on factors such as location, wind patterns, and space constraints.
Offshore wind farm innovations: floating platforms and deepwater installation
Offshore wind farms have opened up vast new possibilities for renewable energy production. Traditional offshore turbines are fixed to the seabed in relatively shallow waters. However, floating platforms are revolutionizing the industry by allowing wind farms to be installed in deeper waters, where wind resources are often stronger and more consistent.
These floating structures are anchored to the seabed but can move slightly with the waves, reducing stress on the turbine components. This innovation has significantly expanded the potential areas for offshore wind development, particularly in regions with deep coastal waters.
Smart grid integration: SCADA systems and real-time monitoring
The integration of wind farms into the power grid has been greatly enhanced by advanced Supervisory Control and Data Acquisition (SCADA) systems. These SCADA
systems provide real-time monitoring and control of wind turbines, allowing operators to optimize performance and quickly respond to changing conditions.
Real-time monitoring enables predictive maintenance, reducing downtime and increasing overall efficiency. It also helps in managing the variable nature of wind power, allowing grid operators to balance supply and demand more effectively.
Advanced materials in turbine design: carbon fiber composites and nano-enhanced coatings
The use of advanced materials has significantly improved the performance and durability of wind turbines. Carbon fiber composites, for instance, are increasingly used in blade construction due to their high strength-to-weight ratio. These lighter blades can be made longer, capturing more wind energy without adding excessive stress to the turbine structure.
Nano-enhanced coatings are another innovation that's making a difference. These coatings protect turbine blades from erosion and ice accumulation, extending their lifespan and maintaining efficiency in harsh environmental conditions. The use of these advanced materials contributes to the overall reliability and cost-effectiveness of wind energy systems.
Grid stability and wind power integration
Integrating large amounts of wind power into existing electrical grids presents unique challenges due to the variable nature of wind. However, significant progress has been made in developing technologies and strategies to ensure grid stability while maximizing the use of wind energy.
Frequency regulation and inertial response of wind turbines
Maintaining a stable grid frequency is crucial for the reliable operation of electrical systems. Traditionally, conventional power plants with large rotating masses provided inertial response to help stabilize frequency. Modern wind turbines are now equipped with advanced control systems that allow them to contribute to frequency regulation and provide synthetic inertia.
These systems enable wind turbines to respond rapidly to grid frequency changes, adjusting their output to help maintain balance. This capability is particularly important as the share of wind power in the energy mix increases, ensuring that the grid remains stable even with high levels of renewable penetration.
Voltage control and reactive power management in wind farms
Voltage stability is another critical aspect of grid operation. Wind farms can now play a significant role in voltage control through sophisticated reactive power management systems. By adjusting the reactive power output of individual turbines, wind farms can help maintain voltage levels within acceptable ranges across the grid.
This capability not only improves overall grid stability but also allows wind farms to provide ancillary services to grid operators, enhancing their value beyond just energy production.
Energy storage solutions: flywheel systems and grid-scale batteries
Energy storage technologies are key to addressing the intermittent nature of wind power. Flywheel systems offer a fast-response solution for short-term energy storage, helping to smooth out rapid fluctuations in wind power output. These systems store energy in the form of rotational kinetic energy and can respond to grid demands within milliseconds.
Grid-scale batteries, particularly lithium-ion batteries, are becoming increasingly important for longer-term energy storage. These batteries can store excess wind energy during high-wind periods and release it when needed, effectively turning wind farms into dispatchable power sources. The combination of wind power and energy storage is transforming the way we think about renewable energy integration.
Forecasting and predictive analytics for wind power generation
Accurate wind power forecasting is essential for efficient grid operation and market integration of wind energy. Advanced forecasting models use a combination of meteorological data, historical performance data, and machine learning algorithms to predict wind power output with increasing accuracy.
These predictive analytics tools allow grid operators to anticipate changes in wind power production and adjust other generation sources accordingly. This improved forecasting capability reduces the need for backup power and helps optimize the overall energy mix, making wind power more reliable and cost-effective.
Environmental and ecological considerations
While wind farms offer significant environmental benefits in terms of clean energy production, their development and operation must be carefully managed to minimize potential negative impacts on local ecosystems.
Avian impact mitigation: radar-assisted curtailment and UV-reflective blades
One of the primary environmental concerns associated with wind farms is their potential impact on bird and bat populations. To address this issue, innovative technologies are being developed and implemented. Radar-assisted curtailment systems use advanced detection algorithms to identify approaching flocks of birds or bats and automatically slow or stop turbine blades to reduce collision risks.
Another promising development is the use of UV-reflective blades. Many birds can see in the ultraviolet spectrum, and coating turbine blades with UV-reflective paint makes them more visible to birds, potentially reducing collision rates. These technologies, combined with careful siting of wind farms away from major migration routes, are helping to minimize the impact on avian populations.
Marine ecosystem effects: artificial reef creation and fish aggregation devices
Offshore wind farms can have both positive and negative effects on marine ecosystems. The foundations of offshore turbines can act as artificial reefs, providing new habitats for marine life. This effect can increase biodiversity in the area and potentially boost local fish populations.
Some offshore wind projects are now incorporating designed structures to enhance this reef effect, creating marine sanctuaries within wind farm areas. Additionally, floating platforms for deepwater wind farms can be equipped with fish aggregation devices, attracting fish and potentially benefiting local fisheries.
Noise reduction technologies: serrated blade edges and active noise control
Noise pollution from wind turbines has been a concern for nearby communities. To address this, manufacturers have developed several noise reduction technologies. Serrated blade edges, inspired by owl feathers, help to reduce the noise produced by turbine blades cutting through the air.
Active noise control systems, similar to those used in noise-canceling headphones, are also being explored for wind turbines. These systems use speakers to emit sound waves that cancel out the noise produced by the turbine. By reducing noise pollution, these technologies help to increase the acceptance of wind farms in local communities.
Economic and policy frameworks for wind energy
The rapid growth of wind energy has been supported by various economic incentives and policy frameworks designed to encourage investment in renewable energy sources. These mechanisms have played a crucial role in making wind power competitive with traditional energy sources.
Production Tax Credits (PTC) and Investment Tax Credits (ITC) for wind projects
In many countries, production tax credits (PTCs) and investment tax credits (ITCs) have been instrumental in driving wind energy development. PTCs provide a tax credit for each kilowatt-hour of electricity generated by a wind farm, while ITCs offer a percentage-based tax credit on the capital investment in wind projects.
These incentives have helped to reduce the overall cost of wind energy, making it more competitive with fossil fuel-based generation. As the cost of wind technology continues to decrease, some countries are phasing out these credits, while others are extending them to support continued growth in the sector.
Power Purchase Agreements (PPAs) and corporate renewable energy procurement
Power Purchase Agreements (PPAs) have become a popular mechanism for financing wind farm projects. These long-term contracts between wind farm operators and electricity buyers (often utilities or large corporations) provide a guaranteed revenue stream for the project, reducing financial risk and making it easier to secure investment.
Corporate renewable energy procurement has also become a significant driver of wind energy development. Many large companies are committing to sourcing 100% of their electricity from renewable sources, often through direct PPAs with wind farms. This trend is creating a robust market for wind energy outside of traditional utility procurement.
Levelized Cost of Energy (LCOE) analysis for wind vs. conventional power sources
The Levelized Cost of Energy (LCOE) is a key metric used to compare the cost-effectiveness of different energy sources. It takes into account the total cost of building and operating a power plant over its lifetime, divided by the total energy output. In recent years, the LCOE of wind energy has fallen dramatically, making it competitive with, and in many cases cheaper than, conventional power sources.
According to recent analyses, onshore wind has become one of the most cost-effective sources of new electricity generation in many parts of the world. Offshore wind, while still more expensive, is also seeing rapid cost reductions as technology improves and economies of scale are realized.
The LCOE of wind energy has decreased by over 70% in the past decade, making it one of the most economically attractive options for new power generation in many markets.
Case studies: successful wind farm integration projects
Examining successful wind farm projects provides valuable insights into the practical application of wind energy technologies and their integration into existing power grids. These case studies demonstrate the scale and impact of modern wind energy projects.
Hornsea Wind Farm: world's largest offshore wind project
The Hornsea Wind Farm, located off the coast of Yorkshire in the UK, is set to become the world's largest offshore wind project when fully completed. The project is being developed in multiple phases, with Hornsea One already operational and Hornsea Two nearing completion.
Hornsea One alone has a capacity of 1.2 GW, powered by 174 turbines, each standing 190 meters tall. The project showcases the latest in offshore wind technology, including advanced foundation designs and high-capacity turbines. It demonstrates the potential for large-scale offshore wind to contribute significantly to national energy supplies.
Gansu Wind Farm: China's onshore wind power megaproject
The Gansu Wind Farm, also known as the Jiuquan Wind Power Base, is a massive onshore wind project in China's Gansu province. It's part of China's ambitious plan to increase its renewable energy capacity. When completed, the project is expected to have a total capacity of 20 GW, making it one of the largest wind farms in the world.
This project highlights the potential for large-scale onshore wind development in regions with favorable wind conditions. It also demonstrates the challenges of integrating massive amounts of wind power into the grid, requiring significant investments in transmission infrastructure.
Hywind Scotland: pioneering floating offshore wind technology
Hywind Scotland, located off the coast of Peterhead, is the world's first commercial-scale floating wind farm. Consisting of five 6 MW turbines, the project demonstrates the viability of floating wind technology for deepwater offshore wind development.
The turbines are mounted on floating structures anchored to the seabed, allowing them to be installed in water depths of up to 800 meters. This technology opens up vast new areas for offshore wind development, particularly in countries with deep coastal waters. The success of Hywind Scotland has paved the way for larger floating wind projects currently in development around the world.
Future trends in wind energy and grid systems
The wind energy sector continues to evolve rapidly, with new technologies and approaches promising to further enhance the efficiency, scalability, and grid integration of wind power. These emerging trends are shaping the future of sustainable energy systems.
Airborne wind energy systems: high-altitude wind kites and tethered drones
Airborne Wind Energy Systems (AWES) represent a radical departure from traditional wind turbine designs. These systems aim to harness the stronger and more consistent winds found at higher altitudes, typically between 200 and 800 meters above ground level.
High-altitude wind kites and tethered drones are two promising AWES technologies. These systems use lightweight flying devices to capture wind energy, either generating electricity onboard or transferring mechanical energy to ground-based generators through a tether. While still in the early stages of development, AWES could potentially access wind resources that are out of reach for conventional turbines, opening up new possibilities for wind energy production.
Machine learning algorithms for turbine performance optimization
Artificial Intelligence (AI) and Machine Learning (ML) are increasingly being applied to optimize wind turbine performance and maintenance. Advanced ML algorithms can analyze vast amounts of data from turbine sensors, weather forecasts, and grid conditions to predict and optimize turbine output.
These systems can adjust turbine parameters in real-time to maximize energy production under varying wind conditions. They can also predict maintenance needs, allowing for proactive repairs and reducing downtime. As these AI systems become more sophisticated, they promise to significantly enhance the efficiency and reliability of wind farms.
Hybrid renewable energy systems: wind-solar-storage integration
The future of renewable energy lies in integrated systems that combine multiple sources of clean power with advanced storage technologies. Hybrid wind-solar-storage systems are gaining traction as a way to provide more consistent and dispatchable renewable energy.
These systems leverage the complementary nature of wind and solar resources – wind often blows stronger at night when solar panels are inactive – and use energy storage to smooth out supply fluctuations. By combining these technologies, hybrid systems can provide a more stable and reliable power supply, potentially replacing conventional baseload power plants.