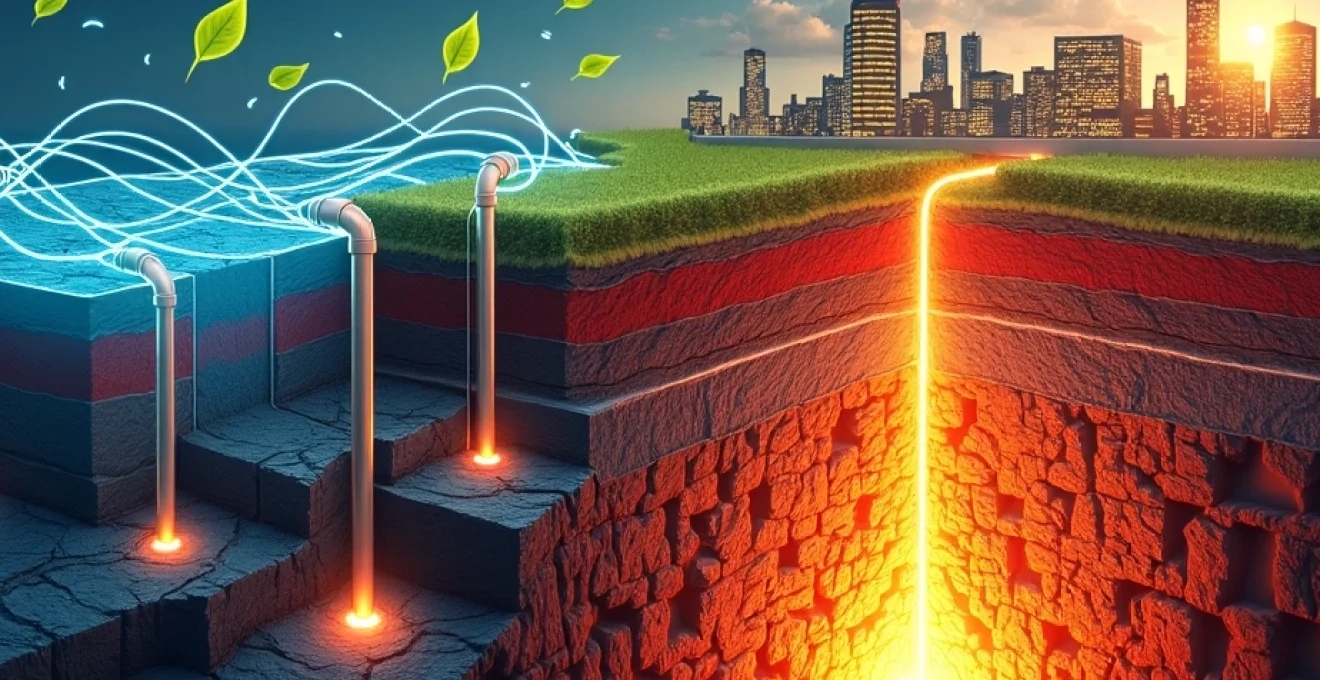
Geothermal energy stands at the forefront of sustainable heating solutions, offering a promising path towards a greener future. As the world grapples with climate change, this renewable resource harnesses the Earth's natural heat to provide clean, reliable power. Recent technological advancements have significantly expanded the potential of geothermal energy, making it more accessible and efficient than ever before.
Enhanced geothermal systems (EGS) for widespread application
Enhanced Geothermal Systems (EGS) represent a groundbreaking approach to harnessing geothermal energy in areas previously deemed unsuitable. Unlike traditional geothermal plants that rely on naturally occurring hot water reservoirs, EGS technology creates artificial reservoirs by injecting water into hot, dry rock formations deep underground. This process, known as hydraulic stimulation, enhances the rock's permeability, allowing for the efficient extraction of heat.
The potential of EGS is enormous, as it significantly expands the geographical scope of geothermal energy production. Regions without conventional geothermal resources can now tap into this clean energy source, reducing their dependence on fossil fuels. Moreover, EGS offers a more stable and predictable power output compared to intermittent renewable sources like wind and solar, making it an invaluable component of a diversified clean energy portfolio.
However, the implementation of EGS is not without challenges. The high initial costs and the need for advanced drilling technologies can be barriers to entry. Additionally, careful management is required to mitigate potential environmental impacts, such as induced seismicity. Despite these hurdles, ongoing research and development are steadily improving the efficiency and safety of EGS, positioning it as a key player in the future of sustainable energy production.
Advanced drilling techniques in geothermal exploration
The success of geothermal energy projects hinges largely on the ability to access heat resources deep within the Earth's crust. Traditional drilling methods often fall short when faced with the extreme temperatures and hard rock formations encountered in geothermal reservoirs. To overcome these challenges, the industry has developed a suite of advanced drilling techniques that are revolutionizing geothermal exploration and development.
Directional drilling with Measurement-While-Drilling (MWD) technology
Directional drilling, coupled with Measurement-While-Drilling (MWD) technology, has significantly enhanced the precision and efficiency of geothermal well construction. This technique allows engineers to steer the drill bit along a predetermined path, accessing geothermal resources that were previously unreachable. MWD systems provide real-time data on the drill bit's position, orientation, and surrounding formation characteristics, enabling on-the-fly adjustments to optimize drilling performance.
The benefits of directional drilling with MWD are manifold. It reduces the number of wells needed to access a geothermal reservoir, minimizing surface disturbance and lowering overall project costs. Furthermore, this technology enables the drilling of multilateral wells from a single surface location, maximizing resource extraction while minimizing environmental impact.
High-temperature resistant drill bits and fluids
The extreme temperatures encountered in geothermal reservoirs pose significant challenges to conventional drilling equipment. To address this, engineers have developed specialized high-temperature resistant drill bits and drilling fluids. These innovations allow for extended drilling operations in environments where temperatures can exceed 300°C (572°F).
Advanced drill bits feature thermally stable polycrystalline diamond compacts (TSP) and other heat-resistant materials that maintain their cutting effectiveness at high temperatures. Similarly, synthetic-based drilling fluids have been formulated to maintain their viscosity and lubricating properties under extreme heat, ensuring efficient debris removal and drill bit cooling.
Plasma drilling for hard rock formations
Plasma drilling represents a cutting-edge technology that holds great promise for geothermal exploration in hard rock formations. This technique uses high-temperature plasma to melt and vaporize rock, creating boreholes without the need for mechanical cutting. Plasma drilling offers several advantages over conventional methods:
- Faster penetration rates in hard rock formations
- Reduced wear on drilling equipment
- Potential for creating smoother wellbores, enhancing fluid flow
- Lower environmental impact due to reduced waste generation
While still in the experimental stage, plasma drilling could revolutionize geothermal exploration by making it feasible to access heat resources in previously impenetrable rock formations.
Thermal spallation drilling in geothermal reservoirs
Thermal spallation drilling is another innovative technique gaining traction in the geothermal industry. This method uses intense heat to cause rapid thermal expansion in the rock, resulting in fractures and spalling. The fractured rock is then easily removed, creating a borehole without the need for conventional mechanical drilling.
The advantages of thermal spallation drilling include:
- Higher penetration rates in hard rock formations
- Reduced equipment wear and lower maintenance costs
- Potential for creating larger diameter boreholes, enhancing geothermal fluid production
As research in this area progresses, thermal spallation drilling may become a game-changer for accessing deep geothermal resources in challenging geological environments.
Binary cycle power plants: maximizing low-temperature resources
Binary cycle power plants have emerged as a crucial innovation in geothermal energy, enabling the exploitation of low-temperature resources that were previously considered uneconomical. These plants utilize a secondary working fluid with a lower boiling point than water to generate electricity, significantly expanding the range of geothermal resources that can be tapped for power production.
Organic Rankine Cycle (ORC) systems for geothermal applications
The Organic Rankine Cycle (ORC) is the most widely used binary cycle system in geothermal power generation. ORC systems employ organic fluids such as pentane or butane, which have lower boiling points than water, allowing for efficient heat exchange with low-temperature geothermal resources. The process works as follows:
- Geothermal fluid transfers heat to the organic working fluid in a heat exchanger
- The vaporized organic fluid drives a turbine to generate electricity
- The organic fluid is then condensed and recycled through the system
- The cooled geothermal fluid is reinjected into the reservoir
ORC systems have proven highly effective in harnessing geothermal resources with temperatures as low as 80°C (176°F), opening up new possibilities for geothermal power generation in regions previously deemed unsuitable.
Kalina Cycle technology in geothermal power generation
The Kalina Cycle represents another innovative approach to binary cycle power generation. This technology uses a mixture of ammonia and water as the working fluid, offering several advantages over traditional ORC systems:
- Higher thermal efficiency, particularly in low-temperature applications
- Better matching of the working fluid's temperature profile to the geothermal resource
- Reduced environmental impact due to the use of non-toxic working fluids
While Kalina Cycle plants are less common than ORC systems, they show great promise for maximizing power output from low-temperature geothermal resources. Ongoing research and development are focused on optimizing the ammonia-water mixture ratios and system configurations to further enhance efficiency.
Working fluid innovations for improved efficiency
The quest for more efficient binary cycle systems has led to significant innovations in working fluid technology. Researchers are exploring novel working fluids and mixtures that offer improved thermodynamic properties and environmental performance. Some promising developments include:
- Zeotropic mixtures that provide better temperature matching during heat exchange
- Supercritical CO2 as a working fluid, offering high efficiency and low environmental impact
- Nanofluids that enhance heat transfer properties
These advancements in working fluid technology have the potential to significantly boost the efficiency of binary cycle power plants, making geothermal energy an even more attractive option for sustainable power generation.
Deep Direct-Use (DDU) geothermal systems for urban heating
Deep Direct-Use (DDU) geothermal systems represent a groundbreaking approach to urban heating, leveraging moderate-temperature geothermal resources to provide sustainable thermal energy for large-scale applications. Unlike traditional geothermal power plants that focus on electricity generation, DDU systems directly utilize geothermal heat for various purposes, including district heating, industrial processes, and even cooling through absorption chiller technology.
The concept of DDU is particularly appealing for urban areas where the demand for heating and cooling is high, and the reduction of carbon emissions is a priority. These systems typically tap into geothermal resources at depths of 1-3 km, where temperatures range from 50°C to 150°C (122°F to 302°F). The extracted heat is then distributed through a network of insulated pipes to end-users, providing a clean and efficient alternative to fossil fuel-based heating systems.
One of the key advantages of DDU geothermal systems is their scalability. They can be designed to serve individual large buildings, such as hospitals or universities, or entire neighborhoods through district heating networks. This flexibility makes DDU an attractive option for urban planners looking to implement sustainable heating solutions at various scales.
Deep Direct-Use geothermal systems have the potential to revolutionize urban heating, offering a sustainable and efficient solution that can significantly reduce a city's carbon footprint.
Moreover, DDU systems can be integrated with other renewable energy sources and energy storage technologies to create comprehensive, resilient energy systems for urban areas. For instance, excess heat from solar thermal systems during summer months can be stored in the geothermal reservoir for use during winter, enhancing the overall efficiency and reliability of the heating system.
Smart geothermal grid integration and management
As geothermal energy plays an increasingly significant role in the global energy mix, the integration and management of geothermal resources within smart grids have become crucial. Smart geothermal grid integration leverages advanced technologies to optimize the production, distribution, and consumption of geothermal energy, enhancing overall system efficiency and reliability.
Load balancing with artificial intelligence in geothermal networks
Artificial Intelligence (AI) is revolutionizing load balancing in geothermal networks. Advanced AI algorithms analyze vast amounts of data from various sources, including weather forecasts, historical consumption patterns, and real-time demand fluctuations, to predict and optimize energy distribution. This predictive load balancing ensures that geothermal energy is utilized efficiently, reducing waste and improving overall system performance.
AI-driven systems can also adapt to unexpected changes in demand or supply, automatically adjusting the output of geothermal plants or redirecting energy flows to maintain grid stability. This level of intelligent management is particularly valuable for integrating geothermal energy with other renewable sources, creating a more resilient and flexible energy infrastructure.
Demand Response systems for geothermal district heating
Demand Response (DR) systems are emerging as a powerful tool for managing geothermal district heating networks. These systems enable real-time communication between energy providers and consumers, allowing for dynamic adjustment of heating supply based on actual demand. In a geothermal context, DR systems can:
- Optimize the distribution of geothermal heat during peak and off-peak hours
- Incentivize consumers to shift their energy usage to off-peak times
- Reduce overall energy consumption through improved efficiency
By implementing DR systems, geothermal district heating networks can achieve better load balancing, reduced operational costs, and improved customer satisfaction. This technology is particularly beneficial in urban areas where heating demands can fluctuate significantly throughout the day.
Blockchain technology in geothermal energy trading
Blockchain technology is poised to transform the way geothermal energy is traded and managed within smart grids. By providing a secure, transparent, and decentralized platform for energy transactions, blockchain can facilitate peer-to-peer energy trading, enabling consumers to buy and sell excess geothermal energy directly.
The benefits of blockchain in geothermal energy trading include:
- Enhanced transparency and security in energy transactions
- Reduced transaction costs and improved efficiency
- Easier integration of small-scale geothermal producers into the grid
- Improved tracking of renewable energy credits and carbon offsets
As blockchain technology matures, it has the potential to create more dynamic and efficient geothermal energy markets, encouraging greater adoption of this sustainable resource.
Real-time monitoring and predictive maintenance of geothermal plants
The implementation of real-time monitoring systems and predictive maintenance strategies is crucial for optimizing the performance and longevity of geothermal power plants. Advanced sensors and Internet of Things (IoT) devices continuously collect data on various operational parameters, including temperature, pressure, flow rates, and equipment performance.
This data is then analyzed using sophisticated algorithms and machine learning models to:
- Detect anomalies and potential equipment failures before they occur
- Optimize maintenance schedules, reducing downtime and costs
- Improve overall plant efficiency through data-driven decision-making
- Extend the lifespan of critical components
By embracing these smart monitoring and maintenance techniques, geothermal plant operators can significantly enhance the reliability and efficiency of their facilities, ensuring a stable supply of clean energy to the grid.
Environmental impact mitigation in geothermal energy production
While geothermal energy is inherently cleaner than fossil fuels, it's not without environmental considerations. As the industry grows, there's an increasing focus on developing technologies and practices to mitigate potential environmental impacts, ensuring that geothermal energy remains a truly sustainable option.
Closed-loop geothermal systems: reducing water consumption
Water consumption has been a significant concern in traditional geothermal systems, particularly in water-scarce regions. Closed-loop geothermal systems address this issue by recirculating the same fluid through the system, dramatically reducing water consumption. These systems use a working fluid, typically a mixture of water and environmentally friendly additives, that is continuously recycled through the heat exchange process.
The benefits of closed-loop systems extend beyond water conservation. They also minimize the risk of groundwater contamination and reduce the potential for induced seismicity associated with fluid injection. As technology advances, we're seeing the development of even more efficient closed-loop systems that can operate at greater depths and in a wider range of geological settings.
CO2 sequestration in geothermal reservoirs
An innovative approach to enhancing the environmental benefits of geothermal energy is the integration of carbon dioxide (CO2) sequestration into geothermal operations. This process involves capturing CO2 from industrial sources and injecting it into geothermal reservoirs, where it serves a dual purpose:
- Enhancing heat extraction by improving fluid circulation in the reservoir
- Permanently storing CO2 underground, reducing greenhouse gas emissions
This technique, known as CO2-Plume Geothermal (CPG), not only boosts the efficiency of geothermal power generation but also contributes to climate change mitigation efforts. Research in this area is ongoing, with pilot projects demonstrating the feasibility and potential of combining geothermal energy production with carbon capture and storage.
Induced seismicity management in EGS projects
One of the primary concerns associated with Enhanced Geothermal Systems (EGS) is the potential for induced seismicity during hydraulic stimulation. To address this issue. Recent advancements in seismic monitoring and prediction technologies have significantly improved our ability to manage and mitigate induced seismicity risks in EGS projects. Some key strategies include:
- Advanced seismic monitoring networks that provide real-time data on micro-seismic events
- Sophisticated algorithms for analyzing seismic patterns and predicting potential larger events
- Adaptive injection protocols that adjust fluid pressures and volumes based on seismic activity
- Traffic light systems that establish clear thresholds for continuing, modifying, or halting operations based on seismic risk
EGS projects can significantly reduce the risk of induced earthquakes while maximizing geothermal resource extraction. This proactive approach not only enhances the safety of geothermal operations but also improves public acceptance and regulatory compliance.
As the geothermal industry continues to evolve, the focus on environmental impact mitigation remains paramount. Through innovative technologies and best practices, geothermal energy is solidifying its position as a truly sustainable and environmentally responsible energy source. The ongoing research and development in areas such as closed-loop systems, CO2 sequestration, and seismic risk management are paving the way for a future where geothermal energy can be harnessed safely and efficiently on a global scale.