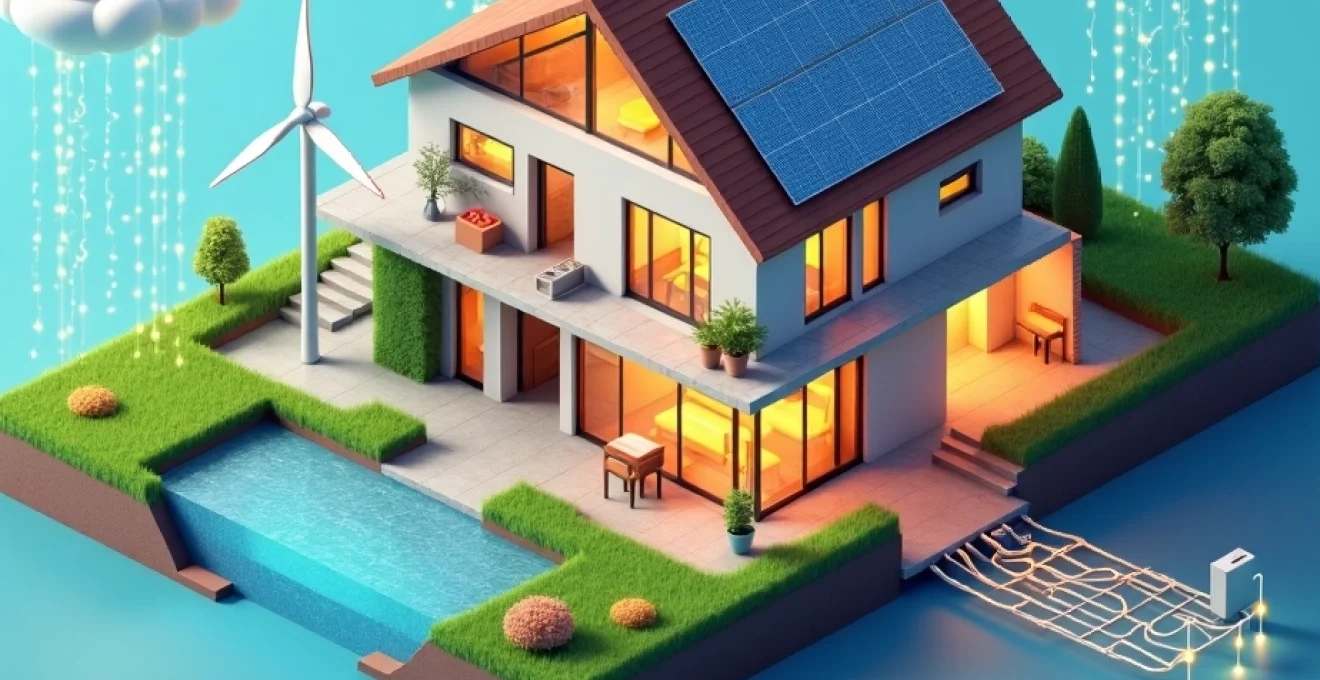
As the world shifts towards sustainable energy practices, homeowners are increasingly exploring small-scale renewable solutions to power their residences. These innovative technologies not only reduce carbon footprints but also offer long-term cost savings and energy independence. From rooftop solar panels to micro wind turbines, the options for residential renewable energy are diverse and rapidly evolving.
Residential solar PV systems: components and installation
Solar photovoltaic (PV) systems have become the cornerstone of residential renewable energy solutions. These systems harness sunlight and convert it into electricity, providing a clean and reliable power source for homes. The core components of a residential solar PV system include solar panels, inverters, mounting systems, and, increasingly, battery storage units. Understanding these elements is crucial for homeowners considering solar energy adoption.
Monocrystalline vs. polycrystalline panels: efficiency comparison
When selecting solar panels, homeowners often face the choice between monocrystalline and polycrystalline technologies. Monocrystalline panels are known for their higher efficiency and sleek appearance, typically converting 20-22% of sunlight into electricity. In contrast, polycrystalline panels offer a more cost-effective solution but with slightly lower efficiency rates of 15-17%. The decision between the two often depends on available roof space, budget constraints, and aesthetic preferences.
Monocrystalline panels are particularly advantageous for homes with limited roof space, as their higher efficiency means more power generation per square foot. However, polycrystalline panels can be an excellent choice for larger installations where cost is a primary concern. It's important to note that both technologies have seen significant improvements in recent years, narrowing the efficiency gap.
Inverter technologies: string, microinverters and power optimizers
Inverters play a crucial role in solar PV systems by converting the direct current (DC) generated by solar panels into alternating current (AC) used in homes. There are three main types of inverter technologies available for residential installations:
- String inverters: The traditional and most cost-effective option, suitable for installations with consistent sunlight exposure.
- Microinverters: Installed on each panel, offering optimized performance for systems with partial shading or complex roof orientations.
- Power optimizers: A hybrid solution that combines string inverter efficiency with panel-level optimization.
The choice of inverter technology can significantly impact system performance and monitoring capabilities. Microinverters and power optimizers, while more expensive, offer panel-level monitoring and can mitigate the effects of shading or panel mismatch, potentially increasing overall system output.
Solar mounting systems: roof types and ground installations
The mounting system is a critical component that secures solar panels to your roof or ground. For roof installations, the type of mounting system depends on the roof material and structure. Pitched roofs typically use rail-based systems, while flat roofs often employ ballasted or penetrating systems. Ground-mounted systems are an alternative for properties with ample land space or unsuitable roof conditions.
When considering roof installations, it's essential to assess the roof's structural integrity and remaining lifespan. Ideally, solar panels should be installed on roofs with at least 15-20 years of life left to avoid the need for reinstallation during the system's lifetime. Ground-mounted systems offer easier access for maintenance but may require additional permits and have higher installation costs.
Battery storage integration to residential solar installations
Battery storage systems are becoming increasingly popular additions to residential solar installations, allowing homeowners to store excess energy for use during peak hours or power outages. Two leading options in the market are the Tesla Powerwall and LG Chem RESU systems. These lithium-ion batteries offer high energy density, long cycle life, and smart energy management features.
The Tesla Powerwall, for instance, boasts a usable capacity of 13.5 kWh and can be stacked for increased storage. The LG Chem RESU series offers various capacities ranging from 9.8 kWh to 16 kWh, catering to different household needs. When integrating battery storage, it's crucial to consider factors such as daily energy consumption, peak usage times, and backup power requirements to size the system appropriately.
Micro wind turbines for domestic power generation
While solar energy dominates the residential renewable energy market, micro wind turbines offer an alternative or complementary solution for homes in windy areas. These small-scale wind energy systems can generate electricity for individual properties, potentially providing significant energy savings over time.
Horizontal axis vs. vertical axis designs for urban settings
Micro wind turbines come in two primary designs: horizontal axis wind turbines (HAWTs) and vertical axis wind turbines (VAWTs). HAWTs are the more traditional design, resembling miniature versions of large-scale wind farm turbines. They are generally more efficient in consistent wind conditions but require precise alignment with the wind direction.
VAWTs, on the other hand, offer several advantages for urban and suburban settings. Their vertical design allows them to capture wind from any direction without needing to reorient, making them more suitable for areas with turbulent or changing wind patterns. VAWTs also tend to be quieter and can be more aesthetically pleasing, which is an important consideration in residential areas.
Wind resource assessment: using anemometers and wind maps
Before installing a micro wind turbine, it's crucial to conduct a thorough wind resource assessment. This process involves measuring the average wind speed and direction at your specific location over an extended period. Anemometers are the primary tools used for this purpose, providing accurate wind speed measurements.
In addition to on-site measurements, wind maps can offer valuable insights into regional wind patterns. These maps, often provided by national weather services or renewable energy organizations, give a broader picture of wind resources in your area. However, local topography and obstacles can significantly affect wind patterns, so site-specific assessments are still necessary for accurate predictions of energy production.
Grid-tie vs. Off-Grid configurations for micro wind systems
Micro wind systems can be configured either as grid-tied or off-grid installations, each with its own set of advantages and considerations. Grid-tied systems allow excess electricity to be fed back into the utility grid, potentially earning credits or payments through net metering programs. These systems also provide the security of grid power when wind resources are insufficient.
Off-grid configurations, typically paired with battery storage, offer energy independence and are ideal for remote locations without access to the utility grid. However, they require careful sizing to ensure sufficient power generation and storage capacity to meet household needs during periods of low wind. Hybrid systems, combining wind with solar and battery storage, can provide a more reliable off-grid solution by diversifying energy sources.
Geothermal heat pumps: harnessing earth's thermal energy
Geothermal heat pumps, also known as ground source heat pumps, offer an efficient and sustainable solution for heating and cooling homes. These systems leverage the constant temperature of the earth below the frost line to transfer heat into or out of a building, depending on the season. With proper installation, geothermal systems can provide significant energy savings and reduced carbon emissions compared to traditional HVAC systems.
Closed-loop vs. open-loop systems: site-specific considerations
Geothermal heat pump systems are primarily categorized into closed-loop and open-loop configurations. Closed-loop systems circulate a heat transfer fluid through a series of buried pipes, exchanging heat with the surrounding soil or rock. These systems are versatile and can be installed in various soil conditions.
Open-loop systems, on the other hand, use groundwater directly as the heat exchange medium. They require a suitable water source, such as a well or pond, and may be subject to additional environmental regulations. While open-loop systems can be more efficient, their feasibility is highly dependent on local water quality and availability.
Vertical vs. horizontal ground loops: space and efficiency factors
For closed-loop systems, the ground loop can be installed either vertically or horizontally, depending on available land space and soil conditions. Vertical loops consist of pipes inserted into deep boreholes, typically 100-400 feet deep. This configuration is ideal for properties with limited land area or rocky soil conditions.
Horizontal loops require more land area but are generally less expensive to install. These systems involve burying pipes in trenches at a depth of 4-6 feet. A variation of the horizontal system is the slinky loop, which coils the pipe to increase heat transfer surface area in a smaller footprint. The choice between vertical and horizontal installations often comes down to a balance of available space, installation costs, and system efficiency.
Geothermal heat pump efficiency: COP and EER ratings explained
The efficiency of geothermal heat pumps is typically measured using two ratings: Coefficient of Performance (COP) for heating and Energy Efficiency Ratio (EER) for cooling. COP represents the ratio of heat output to electrical energy input, with higher values indicating greater efficiency. Most geothermal systems have COPs ranging from 3.0 to 5.0, meaning they produce 3 to 5 units of heat for every unit of electricity consumed.
EER measures cooling efficiency, with higher numbers indicating better performance. Geothermal systems often achieve EER ratings of 15-25, significantly higher than traditional air-source heat pumps. When evaluating geothermal heat pump efficiency, it's important to consider both COP and EER ratings, as well as how they translate to real-world performance in your specific climate and soil conditions.
Micro-hydro power: utilizing on-property water resources
For homeowners with access to flowing water on their property, micro-hydro power systems offer a consistent and reliable source of renewable energy. These systems harness the energy of moving water to generate electricity, providing a continuous power supply that can complement or replace other energy sources.
Impulse vs. reaction turbines for small-scale hydro
Micro-hydro systems typically use one of two types of turbines: impulse or reaction. Impulse turbines, such as Pelton wheels, are ideal for high-head, low-flow situations. They operate by directing a jet of water at buckets mounted around the turbine wheel, efficiently converting the water's kinetic energy into rotational motion.
Reaction turbines, including Francis and Kaplan types, are better suited for low-head, high-flow scenarios. These turbines are immersed in the water flow and rely on both the water's pressure and movement to spin the runner. The choice between impulse and reaction turbines depends on the specific characteristics of your water resource, including head (vertical drop) and flow rate.
Head and flow rate calculations for system sizing
Accurate measurements of head and flow rate are crucial for sizing a micro-hydro system and selecting the appropriate turbine. Head is typically measured in feet or meters and represents the vertical distance the water falls. Flow rate, measured in cubic feet per second or liters per second, indicates the volume of water passing through the system over time.
To calculate potential power output, use the formula:
Power (watts) = Head (feet) × Flow (gpm) × 0.18 efficiency factor
This calculation provides a rough estimate of system capacity, helping homeowners determine if micro-hydro is a viable option for their property. Professional assessments are recommended for precise measurements and system design.
Environmental considerations: fish ladders and water rights
Installing a micro-hydro system requires careful consideration of environmental impacts and legal requirements. Fish ladders or bypass systems may be necessary to allow aquatic life to navigate around the turbine installation. These structures ensure that the micro-hydro system doesn't disrupt local ecosystems or fish migration patterns.
Water rights are another critical factor to consider. In many regions, the use of water for power generation is regulated, and permits may be required. It's essential to research local water rights laws and obtain necessary permissions before proceeding with a micro-hydro installation. Consulting with local environmental agencies and water resource departments can help navigate these requirements and ensure compliance.
Biomass heating systems for residential applications
Biomass heating systems offer a renewable alternative to traditional fossil fuel-based heating, utilizing organic materials as fuel sources. For homeowners, especially those in rural areas with access to wood or agricultural byproducts, biomass can provide an efficient and sustainable heating solution.
Pellet stoves vs. wood gasification boilers
Two popular biomass heating options for residential use are pellet stoves and wood gasification boilers. Pellet stoves burn compressed wood or biomass pellets, offering a convenient and relatively clean-burning option. These stoves typically achieve efficiency rates of 70-83%, making them a highly efficient choice for space heating.
Wood gasification boilers, on the other hand, use a two-stage combustion process to extract maximum energy from wood logs. By gasifying the wood before combustion, these systems can achieve efficiency rates of up to 90%. While more complex and typically more expensive than pellet stoves, wood gasification boilers can provide whole-house heating and hot water, making them suitable for larger homes or those looking to completely replace conventional heating systems.
Feedstock options for biomass heating systems
The choice of feedstock for biomass heating systems depends on local availability, system requirements, and storage capabilities. Wood pellets are a popular option due to their consistency, high energy density, and ease of handling. They are made from compressed sawdust or wood shavings and offer a uniform fuel source that works well in automated feeding systems.
Wood chips present a less processed alternative, often sourced from forestry operations or tree care services. While generally less expensive than pellets, wood chips require more storage space and may have varying moisture content, affecting combustion efficiency. Agricultural residues, such as corn stalks or nut shells, can also serve as biomass fuel in some systems, providing a use for what might otherwise be waste products.
Automated fuel feeding systems and storage solutions
Modern biomass heating systems often incorporate automated fuel feeding mechanisms to enhance convenience and maintain consistent heat output. These systems typically include a storage hopper or silo connected to the boiler or stove via an auger or vacuum system. Automated feeders can be programmed to deliver fuel as needed, reducing the manual labor involved in maintaining the heating system.
Proper storage is crucial for maintaining fuel quality and system efficiency. Wood pellets and chips should be stored in dry, well-ventilated areas to prevent moisture absorption and degradation. Purpose-built storage solutions, such as fabric silos or custom-built sheds, can protect the fuel from the elements while allowing easy access for delivery and feeding systems. When designing storage, consider factors such as fuel type, annual heating requirements, and delivery frequency to ensure adequate capacity and accessibility.
Smart home integration for renewable energy management
As residential renewable energy systems become more sophisticated, integrating them with smart home technologies offers enhanced control, efficiency, and convenience. Smart integration allows homeowners to optimize energy production, storage, and consumption, maximizing the benefits of their renewable energy investments.
Energy monitoring platforms and consumption tracking
Advanced energy monitoring platforms like Sense and Neurio provide real-time insights into household energy consumption and production. These systems use machine learning algorithms to identify individual appliances and devices, offering granular data on energy usage patterns. By integrating with renewable energy systems, these platforms can help homeowners make informed decisions about when to use self-generated power versus grid electricity.
Sense, for example, uses current sensors installed in the electrical panel to monitor energy flow. It can detect subtle changes in electrical signals to identify specific devices, providing detailed energy consumption breakdowns. Neurio offers similar functionality, with the added capability of integrating directly with some solar inverter systems for comprehensive energy production and consumption tracking.
Demand response systems: grid interaction and peak shaving
Smart home systems can enable participation in demand response programs, allowing homeowners to adjust their energy usage based on grid conditions. During periods of high demand, these systems can automatically reduce consumption or switch to stored energy, helping to balance the grid and potentially earning incentives from utility companies.
Peak shaving,
the concept of shifting energy consumption to off-peak hours, can be achieved through smart appliances and energy storage systems. By intelligently managing when high-energy devices operate or when to draw from battery storage, homeowners can reduce strain on the grid and potentially benefit from lower off-peak electricity rates.
Ai-powered energy optimization: machine learning algorithms
Artificial intelligence and machine learning are revolutionizing home energy management. Advanced algorithms can analyze historical energy usage patterns, weather forecasts, and real-time pricing data to optimize renewable energy systems. These AI-powered solutions can predict energy production and consumption, automatically adjusting system settings to maximize efficiency.
For example, machine learning algorithms can forecast solar panel output based on weather predictions and historical performance data. This information can be used to optimize battery charging and discharging cycles, ensuring that stored energy is available when it's most needed or most valuable. Similarly, AI can learn a household's energy consumption patterns and automatically adjust thermostat settings or appliance usage to minimize waste and reduce costs.
IoT devices for renewable energy control: smart thermostats and switches
Internet of Things (IoT) devices play a crucial role in integrating renewable energy systems with smart home technology. Smart thermostats, such as Nest or ecobee, can interface with renewable energy systems to optimize heating and cooling based on energy availability and pricing. These devices learn from user behavior and can adjust temperature settings to maximize comfort while minimizing energy consumption.
Smart switches and plugs enable granular control over individual appliances and devices. When integrated with a home energy management system, these IoT devices can automatically power down non-essential equipment during peak demand periods or when renewable energy production is low. Conversely, they can activate energy-intensive appliances when excess renewable energy is available, ensuring optimal use of self-generated power.
Homeowners can create a synergistic ecosystem where renewable energy sources, storage systems, and household devices work in harmony to maximize efficiency and minimize reliance on the grid. This level of integration not only optimizes energy use but also provides homeowners with unprecedented control and insight into their energy consumption patterns, paving the way for more sustainable and cost-effective home energy management.